Luận án Mô hình đặc tính kênh truyền cho thông tin thủy âm vùng nước nông
Underwater acoustic (UWA) communication systems have been developed for the past three decades [25]. They can be used in potential applications such as environmental monitoring, offshore oil exploration, and
military missions. Nevertheless, UWA communications have a plethora
of difficulties, so they display many challenges for further developments.
The reason can be explained by a large demand on high frequency utilization as well as high data rate access under very complexity shallow
underwater environments. All these requirements, without doubt, call
for intensive research efforts on how to cope with problems faced by
current UWA communications, e.g., limited availability of acoustic frequency spectrum, complex time variations in UWA fading channels, and
urgent needs for good quality of service. Therefore, this dissertation is
devoted to investigate UWA communication systems by considering all
these challenges. In particular, two goals are aimed at, which are known
as: i) UWA channel modeling and ii) performance analysis of UWA
communication systems
Tóm tắt nội dung tài liệu: Luận án Mô hình đặc tính kênh truyền cho thông tin thủy âm vùng nước nông
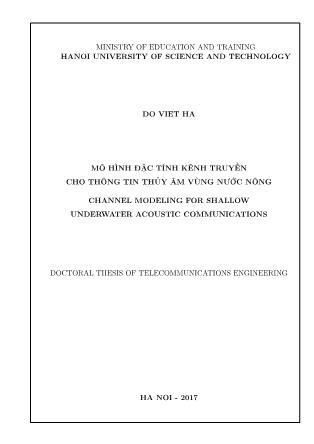
MINISTRY OF EDUCATION AND TRAINING HANOI UNIVERSITY OF SCIENCE AND TECHNOLOGY DO VIET HA MÔ HÌNH ĐẶC TÍNH KÊNH TRUYỀN CHO THÔNG TIN THỦY ÂM VÙNG NƯỚC NÔNG CHANNEL MODELING FOR SHALLOW UNDERWATER ACOUSTIC COMMUNICATIONS DOCTORAL THESIS OF TELECOMMUNICATIONS ENGINEERING HA NOI - 2017 MINISTRY OF EDUCATION AND TRAINING HANOI UNIVERSITY OF SCIENCE AND TECHNOLOGY DO VIET HA MÔ HÌNH ĐẶC TÍNH KÊNH TRUYỀN CHO THÔNG TIN THỦY ÂM VÙNG NƯỚC NÔNG CHANNEL MODELING FOR SHALLOW UNDERWATER ACOUSTIC COMMUNICATIONS Specialization: Telecommunications Engineering Code No: 62520208 DOCTORAL THESIS OF TELECOMMUNICATIONS ENGINEERING SUPERVISORS: 1. Assoc.Prof. Van Duc Nguyen 2. Dr. Van Tien Pham Hanoi - 2017 DECLARATION OF AUTHORSHIP I hereby declare that this dissertation titled, "Channel Modeling for Shal- low Underwater Acoustic Communications”, and the work presented in it are entirely my own original work under the guidance of my supervi- sors. I confirm that: • This work was done wholly or mainly while in candidature for a PhD research degree at Hanoi University of Science and Technology. • Where any part of this dissertation has previously been submitted for a degree of any other qualification at Hanoi University of Science and Technology or any other institution, this has been clearly stated. • Where I have consult the published work or others, this is always given. With the exception of such quotations, this dissertation is entirely my own work. • I have acknowledged all main source of help. • Where the thesis is based on work done by myself jointly with oth- ers, I have made exactly what was done by others and what I have contributed myself. SUPERVISORS Hanoi, August 27, 2017 PhD STUDENT 1. Assoc.Prof. Van Duc Nguyen Do Viet Ha2. Dr. Van Tien Pham ACKNOWLEDGEMENTS First and foremost, I would like to thank my advisor Associate Prof. Dr. Nguyen Van Duc for for providing an excellent atmosphere for doing research, for his valuable comments, constant support and motivation. His guidance helped me in all the time of research and writing of this dissertation. I could not have imagined having a better advisor and mentor for my PhD. I would also like to thank Dr. Pham Van Tien for their advice and feedback, also for many educational and inspiring discussions. My sincere gratitude goes to the members in the Wireless Communica- tion Lab, School of Electronics and Telecommunications, Hanoi Univer- sity of Science and Technology, Hanoi, Vietnam. Without their support and friendship it would have been difficult to complete my PhD studies. I am also thankful to Dr. Nguyen Tien Hoa for his invaluable instruc- tions in presenting my dissertation. I would also like to express my deepest gratitude to my parents, my husband, my son, and my daughter. They were always supporting me and encouraging me with their best wishes, they were standing by me throughout my life. Hanoi, August 27, 2017 PhD STUDENT Do Viet Ha Contents TABLE OF CONTENTS . . . . . . . . . . . . . . . . . . . . . . . . . . . . . . . . . . . . ABBREVIATIONS . . . . . . . . . . . . . . . . . . . . . . . . . . . . . . . . . . . . . . iv LIST OF FIGURES . . . . . . . . . . . . . . . . . . . . . . . . . . . . . . . . . . . . . vi LIST OF TABLES. . . . . . . . . . . . . . . . . . . . . . . . . . . . . . . . . . . . . . . ix INTRODUCTION . . . . . . . . . . . . . . . . . . . . . . . . . . . . . . . . . . . . . . . 1 Chapter 1. DESIGN OF SHALLOW UWA CHANNEL SIMU- LATORS . . . . . . . . . . . . . . . . . . . . . . . . . . . . . . . . . . . . . . . . . . . . . 15 1.1. Introduction . . . . . . . . . . . . . . . . . . . . . . . . . . . . . . . . . . . . . . . . . . . . . . . 16 1.2. Overview of Simulation Models for UWA Channels . . . . . . . . 19 1.2.1. Rayleigh and Rice channels . . . . . . . . . . . . . . . . . . . . . . . . . . . . . 19 1.2.2. Deterministic SOS Channel Models . . . . . . . . . . . . . . . . . . . . . 20 1.2.3. Deterministic SOC Channel Models. . . . . . . . . . . . . . . . . . . . . 21 1.3. The Geometry-based UWA Channel Simulator . . . . . . . . . . . . . 21 1.3.1. Developing the Reference Model from the Geometrical Channel Model . . . . . . . . . . . . . . . . . . . . . . . . . . . . . . . . . . . . . . . . . . . . . . . . . . . . . . . 22 1.3.2. The Simulation Model . . . . . . . . . . . . . . . . . . . . . . . . . . . . . . . . . . 27 1.3.3. The Estimated Parameters of the Simulation Model . . . . 27 1.3.4. Simulation Results . . . . . . . . . . . . . . . . . . . . . . . . . . . . . . . . . . . . . 28 1.4. The Measurement-based UWA Channel Simulator . . . . . . . . . . 28 1.4.1. The Reference Model from the Measurement Data . . . . . . 29 1.4.2. The Simulation Model . . . . . . . . . . . . . . . . . . . . . . . . . . . . . . . . . 32 1.4.3. Estimated Channel Parameters of the Simulation Model 33 1.4.4. Comparison of the Two Channel Simulators . . . . . . . . . . . . 34 1.5. The Proposed Approach for the Static UWA Channel . . . . . . 35 1.5.1. Descriptions . . . . . . . . . . . . . . . . . . . . . . . . . . . . . . . . . . . . . . . . . . . . 36 1.5.2. Results and Discussions . . . . . . . . . . . . . . . . . . . . . . . . . . . . . . . . 38 i ii 1.6. The Proposed Approach for the Case of Doppler Effects . . . . 39 1.6.1. The Measurement Data. . . . . . . . . . . . . . . . . . . . . . . . . . . . . . . . . 40 1.6.2. The Conventional Measurement-based Simulators . . . . . . . 41 1.6.3. The Proposed Channel Simulator . . . . . . . . . . . . . . . . . . . . . . . 45 1.7. Conclusions . . . . . . . . . . . . . . . . . . . . . . . . . . . . . . . . . . . . . . . . . . . . . . . . 50 Chapter 2. MODELING OF DOPPLER POWER SPECTRUM FOR SHALLOW UWA CHANNELS . . . . . . . . . . . . . . . . . . . . 53 2.1. Introduction . . . . . . . . . . . . . . . . . . . . . . . . . . . . . . . . . . . . . . . . . . . . . . . 53 2.2. The Proposed Doppler Spectrum Model . . . . . . . . . . . . . . . . . . . . 56 2.2.1. The Doppler Effects in Shallow UWA Channels . . . . . . . . . 56 2.2.2. The Proposed Doppler Model for UWA Channels . . . . . . . 59 2.3. The Description of Doppler Spectrum Measurements . . . . . . . 63 2.3.1. Experimental Setup . . . . . . . . . . . . . . . . . . . . . . . . . . . . . . . . . . . . 63 2.3.2. Measurement Scenarios . . . . . . . . . . . . . . . . . . . . . . . . . . . . . . . . . 64 2.3.3. Reference Model from the Measurement Data. . . . . . . . . . . 66 2.4. Parameter Optimizations of the Proposed Model . . . . . . . . . . . 67 2.5. Measurement and Doppler Modeling Results . . . . . . . . . . . . . . . 68 2.5.1. Scenario 1 . . . . . . . . . . . . . . . . . . . . . . . . . . . . . . . . . . . . . . . . . . . . . . 69 2.5.2. Scenario 2 . . . . . . . . . . . . . . . . . . . . . . . . . . . . . . . . . . . . . . . . . . . . . . 71 2.5.3. Scenario 3 . . . . . . . . . . . . . . . . . . . . . . . . . . . . . . . . . . . . . . . . . . . . . . 75 2.6. Conclusions . . . . . . . . . . . . . . . . . . . . . . . . . . . . . . . . . . . . . . . . . . . . . . . . 77 Chapter 3. UWA-OFDM SYSTEM PERFORMANCE ANAL- YSIS USING THE MEASUREMENT-BASED UWA CHAN- NEL MODEL. . . . . . . . . . . . . . . . . . . . . . . . . . . . . . . . . . . . . .. . . . 78 3.1. Introduction . . . . . . . . . . . . . . . . . . . . . . . . . . . . . . . . . . . . . . . . . . . . . . . 79 3.2. ICI Analysis of UWA-OFDM Systems . . . . . . . . . . . . . . . . . . . . . . 81 3.2.1. SIR Calculation . . . . . . . . . . . . . . . . . . . . . . . . . . . . . . . . . . . . . . . . 82 3.2.2. Ambient Noise Power . . . . . . . . . . . . . . . . . . . . . . . . . . . . . . . . . . . 83 3.2.3. SINR Calculation. . . . . . . . . . . . . . . . . . . . . . . . . . . . . . . . . . . . . . . 84 3.3. Capacity Calculation . . . . . . . . . . . . . . . . . . . . . . . . . . . . . . . . . . . . . . . 86 iii 3.4. Numerical Results . . . . . . . . . . . . . . . . . . . . . . . . . . . . . . . . . . . . . . . . . . 87 3.4.1. The SIR. . . . . . . . . . . . . . . . . . . . . . . . . . . . . . . . . . . . . . . . . . . . . . . . 88 3.4.2. The SINR . . . . . . . . . . . . . . . . . . . . . . . . . . . . . . . . . . . . . . . . . . . . . . 89 3.4.3. Channel Capacity . . . . . . . . . . . . . . . . . . . . . . . . . . . . . . . . . . . . . . 90 3.4.4. Transmit Power . . . . . . . . . . . . . . . . . . . . . . . . . . . . . . . . . . . . . . . . 92 3.5. Chapter Conclusions . . . . . . . . . . . . . . . . . . . . . . . . . . . . . . . . . . . . . . . 96 CONCLUSIONS . . . . . . . . . . . . . . . . . . . . . . . . . . . . . . . . . . . . . . . . 99 APPENDIX . . . . . . . . . . . . . . . . . . . . . . . . . . . . . . . . . . . . . . . . . . . 103 LIST OF PUBLICATIONS. . . . . . . . . . . . . . . . . . . . . . . . . . . . . 105 ABBREVIATIONS ACF Autocorrelation Function AOA Angles of Arrival AOD Angles of Departure AWGN Additive White Gaussian Noise BPSK Binary Phase Shift Keying CIR Channel Impulse Response FCF Frequency Correlation Function ICI Inter-Channel Interference INLSA Iterative Nonlinear Least Square Approximation ISI Inter-Symbol Interference LNA Low Noise Amplifier LOS Line of Sight LPNM Lp-Norm Method MESS Method of Equally Spaced Scatterers MSE Mean Square Error OFDM Orthogonal Frequency Division Multiplexing PDF Probability Density Function PDP Power Delay Profile PN Pseudo-Noise PSD Power Spectra Density Rx Receiver SINR Signal to Interference plus Noise Ratio SIR Signal-to-Interference Ratio SNR Signal to Noise Ratio SOC Sum-of-Cisoids SOS Sum-of-Sinusoids TCF Time Correlation Function iv vT-FCF Time-Frequency Correlation Function TVCIR Time Variant Channel Impulse Response TVCTF Time-Variant Channel Transfer Function Tx Transmitter UWA Underwater Acoustic WLAN Wireless Local Area Network WSSUS Wide-Sense Stationary Uncorrelated Scattering List of Figures 1 Multipath interference in UWA communication systems. . . . . . . . . . . 4 1.1 The methodology behind the geometry-based channel modelling [17, 55]. . 17 1.2 The methodology behind the measurement-based channel modelling [31, 56]. 18 1.3 The scheme of designing the geometry-based channel simulator [17, 55]. . . 22 1.4 The geometrical model for shallow UWA channels with randomly dis- tributed scatterers Si,n (•) on the surface (i = 1) and the bottom (i = 2) [55]. . . . . . . . . . . . . . . . . . . . . . . . . . . . . . . . . . . . . . . . 23 1.5 The comparison between the normalized FCF of the reference model and that obtained by the geometry-based simulator. . . . . . . . . . . . . . . . 29 1.6 Illustration of the measurement setup in Halong bay. . . . . . . . . . . . . 30 1.7 The measured |hˆ(τ, t)|2 for the transmission distance of 150 m. . . . . . . 31 1.8 The measured and normalized PDP ρ(τ) obtained for the transmission distance of 150 m. . . . . . . . . . . . . . . . . . . . . . . . . . . . . . . . 32 1.9 The comparison of the normalized FCF obtained by the two simulators to that of the reference model. . . . . . . . . . . . . . . . . . . . . . . . . . 35 1.10 The flowchart of proposed approach to design the static UWA channel simulator. . . . . . . . . . . . . . . . . . . . . . . . . . . . . . . . . . . . . 36 1.11 The comparison between the normalized FCF of the reference model and that obtained by the measurement-based, the geometry-based, and the proposed simulators. . . . . . . . . . . . . . . . . . . . . . . . . . . . . . . 38 1.12 The normalized Doppler power spectrum. . . . . . . . . . . . . . . . . . . . 41 1.13 a) The reference T-FCF derived from the measurement results. b) The T-FCF of the channel simulation model designed by the conventional simulator. . . . . . . . . . . . . . . . . . . . . . . . . . . . . . . . . . . . . 43 1.14 The comparison between the normalized T-FCF of the reference model and that obtained by the conventional measurement-based simulator. . . . 44 1.15 The flowchart of the proposed approach for the case of moving Rx. . . . . 46 vi vii 1.16 a) The reference T-FCF derived from the measurement results. b) The T-FCF of the channel simulation model designed by the proposed simulator. 48 1.17 The comparison between the normalized T-FCF of the reference model and that obtained by the proposed simulator. . . . . . . . . . . . . . . . . 49 1.18 a) The error of the simulation T-FCF designed by the conventional measurement-based simulator. b)The error of the simulation T-FCF designed by the proposed simulator. . . . . . . . . . . . . . . . . . . . . . . 51 2.1 The 3-D geometry model for shallow environments with randomly dis- tributed scatterers Si,n (•) on the surface (i = 1) and the bottom (i = 2). . 57 2.2 The Spike-shape Doppler spectrum. . . . . . . . . . . . . . . . . . . . . . . 61 2.3 Effect of the two Doppler components on the overall Doppler spectrum. . . 62 2.4 Illustration of the measurement setup in Halong bay. . . . . . . . . . . . . 64 2.5 The Doppler measurement scenario 1. . . . . . . . . . . . . . . . . . . . . . 64 2.6 The Doppler measurement scenario 2. . . . . . . . . . . . . . . . . . . . . . 65 2.7 The Doppler measurement scenario 3. . . . . . . . . . . . . . . . . . . . . . 65 2.8 The steps of parameter computations. . . . . . . . . . . . . . . . . . . . . . 68 2.9 The reference model S˜n (f) compared with the proposed Doppler model S (f) for four observed cases in scenario 1. . . . . . . . . . . . . . . . . . . 73 2.10 The reference model S˜n (f) compared with the proposed Doppler model S (f) for six typical cases in scenario 2. . . . . . . . . . . . . . . . . . . . . 74 2.11 The reference model S˜n (f) compared with the proposed Doppler model S (f) for six cases in scenario 3. . . . . . . . . . . . . . . . . . . . . . . . . 76 2.12 The estimated trajectory movement of the Rx for scenario 3. . . . . . . . . 77 3.1 Average SIR versus signal bandwidth for different numbers of sub-carriers.. 88 3.2 Average SINR versus signal bandwidth for different numbers of sub-carriers. 89 3.3 Capacity of UWA-OFDM system versus signal bandwidth for different numbers of sub-carriers. . . . . . . . . . . . . . . . . . . . . . . . . . . . . 91 3.4 Average spectra efficiency versus signal bandwidth for the number of sub-carriers N = 256, and SNR = 20 dB at the receiver. . . . . . . . . . . . 92 3.5 Required transmit power PT versus signal bandwidth to achieve an SNR of 20 dB at the receiver. . . . . . . . . . . . . . . . . . . . . . . . . . . . . 93 3.6 Average spectra efficiency versus SNR at the receiver for the number of sub-carriers N = 256, and signal bandwidth B = 10 kHz. . . . . . . . . . . 94 viii 3.7 Average SIR, SINR versus SNR at the receiver for the number of sub- carriers N = 256, and signal bandwidth B = 10 kHz. . . . . . . . . . . . . . 94 3.8 Average spectra efficiency versus SNR at t ... c communications. In 2012 IEEE International Con- ference on Communications (ICC), pp. 3811–3815. IEEE. [28] Deane, G. B., J. C. Preisig, and A. C. Lavery (2013). The suspension of large bubbles near the sea surface by turbulence and their role in absorbing forward- scattered sound. IEEE Journal of Oceanic Engineering 38 (4), 632–641. [29] Dol, H., M. Colin, M. Ainslie, P. van Walree, and J. Janmaat (2013). Simulation of an underwater acoustic communication channel characterized by wind-generated surface waves and bubbles. Oceanic Engineering, IEEE Journal of 38 (4), 642–654. [30] Esmaiel, H. and D. Jiang (2013). Review article: Multicarrier communication for underwater acoustic channel. Int. J. Communications, Network and System Sci- ences 6, 361–376. 109 [31] Fayziyev, A., M. Paetzold, E. Masson, Y. Cocheril, and M. Berbineau (2014). A measurement-based channel model for vehicular communications in tunnels. In Wireless Communications and Networking Conference (WCNC), 2014 IEEE, pp. 116–121. IEEE. [32] Flatte, S. M. (1983). Wave propagation through random media: Contributions from ocean acoustics. Proceedings of the IEEE 71 (11), 1267–1294. [33] Fleury, B. H., M. Tschudin, R. Heddergott, D. Dahlhaus, and K. I. Pedersen (1999). Channel parameter estimation in mobile radio environments using the SAGE algorithm. IEEE Journal on Selected Areas in Communications 17 (3), 434–450. [34] Fuhl, J., J.-P. Rossi, and E. Bonek (1997). High-resolution 3-D direction-of-arrival determination for urban mobile radio. IEEE Transactions on Antennas and Propa- gation 45 (4), 672–682. [35] Hamdi, K. A. (2010). Exact SINR analysis of wireless OFDM in the presence of carrier frequency offset. IEEE Transactions on Wireless Communications 9 (3). [36] Hashemi, H. (1993). The indoor radio propagation channel. Proceedings of the IEEE 81 (7), 943–968. [37] Heitsenrether, R. M., M. Badiey, M. B. Porter, M. Siderius, and W. A. Kuperman (2004). Modeling acoustic signal fluctuations induced by sea surface roughness. In AIP Conference Proceedings, Volume 728, pp. 214–221. AIP. [38] Hogstad, B. O., C. A. Gutie´rrez, M. Pa¨tzold, and P. M. Crespo (2013). Classes of sum-of-cisoids processes and their statistics for the modeling and simulation of mobile fading channels. EURASIP Journal on Wireless Communications and Net- working 2013 (1), 1–15. [39] Ijaz, S., A. J. Silva, O. C. Rodr´ıguez, and S. M. Jesus (2011). Doppler domain decomposition of the underwater acoustic channel response. In OCEANS, 2011 IEEE-Spain, pp. 1–7. IEEE. [40] Iskander, C.-D. and P. T. Mathiopoulos (2005). Analytical envelope correlation and spectrum of maximal-ratio combined fading signals. IEEE transactions on ve- hicular technology 54 (1), 399–404. [41] Jornet, J. M. and M. Stojanovic (Sep. 2008.). Distributed power control for un- derwater acoustic networks. in Proc. OCEANS 2008, Quebec City, Canada. 110 [42] Kim, B. C. and I. Lu (Sep. 2000). Parameter study of OFDM underwater com- munications system. in Proc. IEEE OCEANS 2000, vol. 2, Providence, RI, USA, pp. 1251–1255 . [43] Kunisch, J. and J. Pamp (2008). Wideband car-to-car radio channel measurements and model at 5.9 ghz. In Vehicular Technology Conference, 2008. VTC 2008-Fall. IEEE 68th, pp. 1–5. IEEE. [44] Lasota, H. and I. Kochan´ska (2011). Transmission parameters of underwater com- munication channels. Hydroacoustics 14, 119–126. [45] Lee, P., J. Barter, K. Beach, E. Caponi, C. Hindman, B. Lake, H. Rungaldier, and J. Shelton (1995). Power spectral lineshapes of microwave radiation backscattered from sea surfaces at small grazing angles. In IEE Proceedings-Radar, Sonar and Navigation, Volume 142, pp. 252–258. IET. [46] Li, J. and M. Kavehrad (Dec. 1999.). Effects of time selective multipath fading on OFDM systems for broadband mobile applications. IEEE Communications Letters, vol. 3, no.12, pp. 332–334 . [47] Li, Y. and L. J. Cimini (2001). Bounds on the interchannel interference of OFDM in time-varying impairments. IEEE Transactions on Communications, vol. 49, no. 3, pp. 401–404 . [48] Liu, C., Y. V. Zakharov, and T. Chen (2012). Doubly selective underwater acous- tic channel model for a moving transmitter/receiver. Vehicular Technology, IEEE Transactions on 61 (3), 938–950. [49] Lucani, D. E., M. Stojanovic, and M. Me´dard (2008). On the relationship between transmission power and capacity of an underwater acoustic communication channel. In OCEANS 2008-MTS/IEEE Kobe Techno-Ocean, pp. 1–6. IEEE. [50] Luong, N. S., M. Pa¨tzold, et al. (2017). A method to estimate the path gains and propagation delays of underwater acoustic channels using the arrival phase informa- tion of the multipath components. AEU-International Journal of Electronics and Communications . [51] Maurer, J., T. Fugen, and W. Wiesbeck (2002). Narrow-band measurement and analysis of the inter-vehicle transmission channel at 5.2 ghz. In Vehicular Technology Conference, 2002. VTC Spring 2002. IEEE 55th, Volume 3, pp. 1274–1278. IEEE. 111 [52] McDonald, J. and P. Schultheiss (1975). Asymptotic frequency spread in surface- scatter channels at large Rayleigh numbers. The Journal of the Acoustical Society of America 57 (1), 160–164. [53] Models, T. C. IEEE std. 802.11–03/940r4, May, 2004. [54] Molisch, A. F., F. Tufvesson, J. Karedal, and C. F. Mecklenbrauker (2009). A survey on vehicle-to-vehicle propagation channels. IEEE Wireless Communica- tions 16 (6), 12–22. [55] Naderi, M., M. Paetzold, and A. G. Zajic (2014). A geometry-based channel model for shallow underwater acoustic channels under rough surface and scattering condi- tions. in Proc. 5th International Conference on Communication and Electronics, ICCE 2014. Da Nang City, Vietnam, 112–117. [56] Naderi, M., M. Paetzold, and A. G. Zajic (2015). The design of measurement-based underwater acoustic channel simulators using the INLSA algorithm. In OCEANS 2015-Genova, pp. 1–6. IEEE. [57] Nouri, H., M. Uysal, E. Panayirci, and H. Senol (2014). Information theoretical performance limits of single-carrier underwater acoustic systems. IET Communica- tions 8 (15), 2599–2610. [58] Otnes, R., P. A. van Walree, and T. Jenserud (2013). Validation of replay-based underwater acoustic communication channel simulation. IEEE Journal of Oceanic Engineering 38 (4), 689–700. [59] Paetzold, M., U. Killat, F. Laue, and Y. Li (1998). On the statistical properties of deterministic simulation models for mobile fading channels. IEEE Transactions on Vehicular Technology 47 (1), 254–269. [60] Papoulis, A. and S. U. Pillai (2002). Probability, random variables, and stochastic processes. Tata McGraw-Hill Education. [61] Parkins, B. (1971). Reflection and scattering from a time-varying rough surface– the nearly complete Lloyd’s mirror effect. The Journal of the Acoustical Society of America 49 (5B), 1484–1490. [62] Pa¨tzold, M. (2011). Mobile radio channels. John Wiley & Sons. 112 [63] Pa¨tzold, M., U. Killat, F. Laue, and Y. Li (1996). A new and optimal method for the derivation of deterministic simulation models for mobile radio channels. In Vehicular Technology Conference, 1996. Mobile Technology for the Human Race., IEEE 46th, Volume 3, pp. 1423–1427. IEEE. [64] Pa¨tzold, M., U. Killat, F. Laue, and Y. Li (1998). On the statistical properties of deterministic simulation models for mobile fading channels. IEEE Transactions on Vehicular Technology, 47 (1), 254–269. [65] Patzold, M. and V. Nguyen (2004). A spatial simulation model for shadow fading processes in mobile radio channels. In Personal, Indoor and Mobile Radio Commu- nications, 2004. PIMRC 2004. 15th IEEE International Symposium on, Volume 3, pp. 1832–1838. IEEE. [66] Pa¨tzold, M. and N. Youssef (2015). Spectrogram analysis of multipath fading channels. In Personal, Indoor, and Mobile Radio Communications (PIMRC), 2015 IEEE 26th Annual International Symposium on, pp. 2214–2219. IEEE. [67] Pham, V.-H., M. H. Taieb, J.-Y. Chouinard, S. Roy, and H.-T. Huynh (2011). On the double Doppler effect generated by scatterer motion. REV-JEC (ISSN: 1859- 378X) 1 (1). [68] Polprasert, C., J. Ritcey, M. Stojanovic, et al. (2011). Capacity of OFDM sys- tems over fading underwater acoustic channels. Oceanic Engineering, IEEE Journal of 36 (4), 514–524. [69] Porter, M. et al. Bellhop code.[online] available:. hlsresearch. com. Rays/index. html . [70] Preisig, J. (2007). Acoustic propagation considerations for underwater acoustic communications network development. ACM SIGMOBILE Mobile Computing and Communications Review 11 (4), 2–10. [71] Price, R. (1955). A note on the envelope and phase-modulated components of narrow-band gaussian noise. IRE Transactions on Information Theory 1 (2), 9–13. [72] Proakis, J. and M. Salehi (2008). Digital Communications. McGraw-Hill Interna- tional Edition. McGraw-Hill. 113 [73] Qarabaqi, P. and M. Stojanovic (2009). Statistical modeling of a shallow wa- ter acoustic communication channel. In Proc. Underwater Acoustic Measurements Conference, Nafplion, Greece, pp. 1341–1350. Citeseer. [74] Qarabaqi, P. and M. Stojanovic (2011). Modeling the large scale transmission loss in underwater acoustic channels. In 49th Annual Allerton Conference on Communi- cation, Control, and Computing (Allerton), 2011, pp. 445–452. IEEE. [75] Qarabaqi, P. and M. Stojanovic (2013). Statistical characterization and computa- tionally efficient modeling of a class of underwater acoustic communication channels. IEEE J. Ocean. Eng. 38 (4), 701–717. [76] Radosevic, A., J. G. Proakis, and M. Stojanovic (2009). Statistical characterization and capacity of shallow water acoustic channels. In OCEANS 2009-EUROPE, pp. 1–8. IEEE. [77] Raynal, A. M. and A. W. Doerry (2010). Doppler characteristics of sea clutter. New Mexico: Sandia National Laboratories . [78] Rice, S. O. (1944). Mathematical analysis of random noise. Bell Labs Technical Journal 23 (3), 282–332. [79] Rice, S. O. (1945). Mathematical analysis of random noise. Bell System Technical Journal 24 (1), 46–156. [80] Sehgal, A., I. Tumar, and J. Schonwalder (2009). Variability of available capacity due to the effects of depth and temperature in the underwater acoustic communica- tion channel. In OCEANS 2009-EUROPE, pp. 1–6. IEEE. [81] Sen, I. and D. W. Matolak (2008). Vehicle–vehicle channel models for the 5-ghz band. IEEE Transactions on Intelligent Transportation Systems 9 (2), 235–245. [82] Siderius, M. and M. B. Porter (2008). Modeling broadband ocean acoustic trans- missions with time-varying sea surfaces. The Journal of the Acoustical Society of America 124 (1), 137–150. [83] Socheleau, F.-X., C. Laot, and J.-M. Passerieux (2011). Stochastic replay of non- wssus underwater acoustic communication channels recorded at sea. IEEE Transac- tions on Signal Processing 59 (10), 4838–4849. 114 [84] Socheleau, F.-X., C. Laot, and J.-M. Passerieux (2015). Parametric replay-based simulation of underwater acoustic communication channels. IEEE Journal of Oceanic Engineering 40 (4), 796–806. [85] Socheleau, F.-X., J.-M. Passerieux, and C. Laot (2009). Characterisation of time- varying underwater acoustic communication channel with application to channel capacity. In Underwater Acoustic Measurements. [86] Socheleau, F.-X., M. Stojanovic, C. Laot, and J.-M. Passerieux (2012). Information-theoretic analysis of underwater acoustic OFDM systems in highly dis- persive channels. Journal of Electrical and Computer Engineering, vol. 2012 . [87] Stojanovic, M. (October 2007). On the relationship between capacity and dis- tance in an underwater acoustic communication channel. ACM SIGMOBILE Mobile Computing and Communications Review (MC2R), vol.11, no. 4, pp. 34–43 . [88] Stojanovic, M. and J. Preisig (2009). Underwater acoustic communication chan- nels: Propagation models and statistical characterization. IEEE Communications Magazine 47 (1), 84–89. [89] Tomasi, B., G. Zappa, K. McCoy, P. Casari, and M. Zorzi (2010). Experimental study of the space-time properties of acoustic channels for underwater communica- tions. In OCEANS 2010 IEEE-Sydney, pp. 1–9. IEEE. [90] Tsimenidis, C., B. Sharif, O. Hinton, and A. Adams (2005). Analysis and modelling of experimental doubly-spread shallow-water acoustic channels. In Oceans 2005- Europe, Volume 2, pp. 854–858. [91] Tuteur, F., H. Tung, and J. Zornig (1980). Asymmetric doppler amplitudes in the surface scatter channel for crosswind transmitter–receiver geometry. The Journal of the Acoustical Society of America 68 (4), 1184–1192. [92] Urick, R. J. (1967). Principles of underwater sound for engineers. Tata McGraw- Hill Education. [93] Van Walree, P. (2011). Channel sounding for acoustic communications: techniques and shallow-water examples. Norwegian Defence Research Establishment (FFI), Tech. Rep. FFI-rapport 7. 115 [94] Van Walree, P., T. Jenserud, and R. Otnes (2010). Stretched-exponential doppler spectra in underwater acoustic communication channels. The Journal of the Acous- tical Society of America 128 (5), EL329–EL334. [95] Van Walree, P., T. Jenserud, M. Smedsrud, et al. (2008). A discrete-time channel simulator driven by measured scattering functions. IEEE Journal on Selected Areas in Communications 26 (9), 1628–1637. [96] Van Walree, P., R. Otnes, et al. (2013). Ultrawideband underwater acoustic com- munication channels. IEEE Journal of Oceanic Engineering 38 (4), 678–688. [97] Van Walree, P. A. (2013). Propagation and scattering effects in underwater acous- tic communication channels. IEEE Journal of Oceanic Engineering 38 (4), 614–631. [98] Walker, D. (2000). Experimentally motivated model for low grazing angle radar doppler spectra of the sea surface. IEE Proceedings-Radar, Sonar and Naviga- tion 147 (3), 114–120. [99] Walker, D. (2001). Doppler modelling of radar sea clutter. IEE Proceedings-Radar, Sonar and Navigation 148 (2), 73–80. [100] Ward, K. D., S. Watts, and R. J. Tough (2006). Sea clutter: scattering, the K distribution and radar performance, Volume 20. IET. [101] Watts, S., L. Rosenberg, S. Bocquet, and M. Ritchie (2016). Doppler spectra of medium grazing angle sea clutter; part 1: characterisation. Radar, Sonar Navigation, IET 10 (1), 24–31. [102] Yin, X. and X. Cheng (2016). Propagation channel characterization, parameter estimation, and modeling for wireless communications. John Wiley & Sons. [103] Zajic, A. G. (2010). Statistical modeling of underwater wireless channels. In GLOBECOM, pp. 1–5. [104] Zajic´, A. G. (2011). Statistical modeling of MIMO mobile-to-mobile underwater channels. IEEE Transactions on Vehicular Technology 60 (4), 1337–1351. [105] Zhang, J., J. Cross, and Y. R. Zheng (2010). Statistical channel modeling of wireless shallow water acoustic communications from experiment data. In Military Communications Conference, MILCOM 2010, pp. 2412–2416. IEEE.
File đính kèm:
luan_an_mo_hinh_dac_tinh_kenh_truyen_cho_thong_tin_thuy_am_v.pdf
2.Tom tat luan an_English.pdf
2.Tom tat luan an_Vietnamese.pdf
3. Ban trich yeu luan an.pdf
4. INFORMATION ON NEW CONCLUSIONS OF DOCTORAL THESIS.pdf
5. THÔNG TIN TÓM TẮT VỀ NHỮNG KẾT LUẬN MỚI CỦA LUẬN ÁN TIẾN SĨ (VIỆT).pdf