The effects of elevated temperature and hypoxia on the respiratory organs of pangasianodon hypophthalmus
It has been predicted that the environmental temperature is increasing as a result of
global climate change (IPPC, 2014). It is clear that the projected increase in environmental
temperature may lead to negative effects on fish populations as a result of disturbances on fish
physiology, metabolism, immune functionality, reproductive capacity, behavior, growth
performance, or mortality (Watts et al., 2001; Cnaani, 2006; Dalvi el al., 2009; khoshnevis
Yazdi and Shakouri, 2010; Singh et al., 2013; Reid et al., 2015). Temperature elevation causes
a reduction in oxygen concentration in water (hypoxia), and at the same time an increase in the
metabolic demand for oxygen by living organisms, which has been argued to lead to aerobic
scope reductions. These are the features underlying the oxygen- and capacity-limited thermal
tolerance (OCLTT) hypothesis, which proposes that oxygen delivery is the key mechanism
underlying the negative effects of increased temperatures in aquatic organisms (Pörtner, 2001;
Pörtner and Farrell, 2008; Pörtner, 2010). It has also been noted that elevated temperature
interacting with hypoxia could result in larger effects on organism performance (McBryan et
al., 2013). Tropical regions are predicted to be seriously affected by the climate change. Indeed,
there are more than 7000 freshwater fish species living in tropical regions, and it has been
argued that since these animals are likely stenothermal and since they already live closer to
their upper thermal limit, they may actually be the most vulnerable (Nelson, 1994; Tewksbury
et al., 2008). Besides, tropical areas are known for significant fluctuations in oxygen content
in the water, where water can be richly oxygenated in wet seasons and severely hypoxic or
even anoxic in dry season (Welcomme, 1979; Lucas and Baras, 2008; Nguyễn Lâm Anh,
2016). In addition, as a result of primary production in organically rich water with poor mixing,
oxygen can fluctuate on a dual basis from deep hypoxia at night to hyperoxia during the day.
Tóm tắt nội dung tài liệu: The effects of elevated temperature and hypoxia on the respiratory organs of pangasianodon hypophthalmus
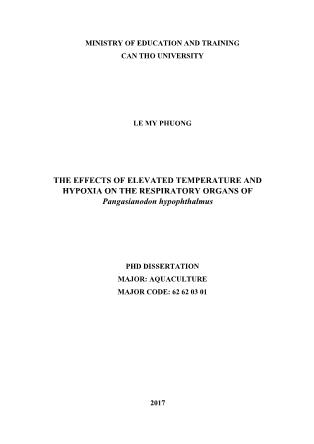
MINISTRY OF EDUCATION AND TRAINING CAN THO UNIVERSITY LE MY PHUONG THE EFFECTS OF ELEVATED TEMPERATURE AND HYPOXIA ON THE RESPIRATORY ORGANS OF Pangasianodon hypophthalmus PHD DISSERTATION MAJOR: AQUACULTURE MAJOR CODE: 62 62 03 01 2017 MINISTRY OF EDUCATION AND TRAINING CAN THO UNIVERSITY LE MY PHUONG THE EFFECTS OF ELEVATED TEMPERATURE AND HYPOXIA ON THE RESPIRATORY ORGANS OF Pangasianodon hypophthalmus PHD DISSERTATION MAJOR: AQUACULTURE MAJOR CODE: 62 62 03 01 SUPERVISORS Supervisor: Assoc. Prof. Dr. DO THI THANH HUONG Co-supervisor: Assoc. Prof. Dr. MARK BAYLEY 2017 1 Data sheet Title: The effects of elevated temperature and hypoxia on the respiratory organs of Pangasianodon hypophthalmus Subtitle: PhD Dissertation Author: Le My Phuong Affiliation: College of Aquaculture & Fisheries, Can Tho University, Vietnam; Zoophysiology, Department of Bioscience, Aarhus University, Denmark. Publication year: 2017 Cite as: Phuong L.M. (2017) The effects of elevated temperature and hypoxia on the respiratory organs of Pangasianodon hypophthalmus. PhD Dissertation. College of Aquaculture & Fisheries, Can Tho University, Vietnam and Zoophysiology, Department of Bioscience, Aarhus University, Denmark. Keywords: climate change, hypoxia, temperature, morphometric, swim bladder, gill remodelling, air-breathing fish, Pangasianodon hypophthalmus Supervisor: Associate Professor Do Thi Thanh Huong, Deparment of Aquatic Nutrition and Products Processing, College of Aquaculture and Fisheries, Can Tho University, Vietnam. Co-supervisor: Associate Professor Mark Bayley, Zoophysiology, Deparment of Bioscience, Aarhus University, Denmark. Co-supervisor: Professor Jens Randel Nyengaard, Core Center for Molecular Morphology, Section for Stereology and Microscopy, Centre for Stochastic Geometry and Advanced Bioimaging, Aarhus University, Denmark. 2 Table of Contents Data sheet .......................................................................................................................... 1 Table of Contents ............................................................................................................. 2 ACKNOWLEDGEMENTS ............................................................................................ 3 SUMMARY ...................................................................................................................... 4 Chapter 1: INTRODUCTION ........................................................................................ 6 1.1. Oxygen requirement and adaptive mechanisms of fish to hypoxia ........................ 6 1.2. Striped catfish (Pangasianodon hypophthalmus) ................................................... 7 1.3. General gill structure in teleosts .............................................................................. 8 1.4. Osmo-respiratory compromise in fish gills ........................................................... 11 1.5. Gill plasticity and environmental factors causing gill plasticity ........................... 12 1.6. Methods applied in morphometric studies ............................................................ 14 1.7. Objectives of dissertation......17 REFERENCES ............................................................................................................. 17 Chapter 2 (PAPER 1) .................................................................................................... 26 Recovery of blood gases and haematological parameters upon anaesthesia with Benzocaine, MS-222 or Aqui-S in the air-breathing catfish (Pangasianodon hypophthalmus) ............................................................................................................... 26 Chapter 3 (PAPER 2) .................................................................................................... 48 Gill remodelling and growth rate of striped catfish Pangasianodon hypophthalmus under impacts of hypoxia and temperatures ............................................................... 48 Chapter 4 (PAPER 3) .................................................................................................... 76 Ontogeny and morphometric of the gill and swim bladder of air-breathing striped catfish Pangasianodon hypophthalmus ............................................................ 76 Chapter 5 (PAPER 4) .................................................................................................. 104 Gill remodelling does not affect the rate of acid-base regulation in the striped catfish Pangasianodon hypophthalmus ....................................................................... 104 Chapter 6: Conclusions and Perspectives. ............................................................. 121 APPENDICE.....125 3 ACKNOWLEDGEMENTS Foremost, I would also like to give my thanks to Assoc. Prof. Do Thi Thanh Huong and Prof. Nguyen Thanh Phuong in Can Tho University for always supporting, encouraging, and watching my steps during my study. I would also like to express my gratitude to Assoc. Prof. Mark Bayley who has found and built my passions and directions for science as who I am and what I have today. His guidance and enthusiasm have inspired and supported me moving forward and working by my best during my research and writing this thesis. I am also grateful to Prof. Jens Randel Nyengaard for teaching me stereological methods and supporting me during my working in his laboratory. My sincere thanks also go to Prof. Tobias Wang, Prof. Atsushi Ishimatsu, and Prof. Hans Malte for giving me special advices and precious and initiative suggestions which contribute importantly in my thesis. Also, I acknowledge Christian Damsgaard who greatly contributed in interpreting and discussing data of the last manuscript in this thesis. I would like to thank the staffs at the College of Aquaculture and Fisheries, Can Tho University (Vietnam), at the Zoophysiology Section, Department of Biological Sciences, and at Core Center for Molecular Morphology, Section for Stereology and Microscopy, Centre for Stochastic Geometry and Advanced Bioimaging, Aarhus University, Denmark where my projects were carried out. Especially, I would like to thank Maj-Britt Lundorf for teaching me with helpful skills in histology and Per Guldhammer Henriksen for helping me during my experiments in Aarhus University. I would also like to thank my fellow friends in iAQUA project: Nguyen Thi Kim Ha, Le Thi Hong Gam, Dang Diem Tuong, and Phan Vinh Thinh for their friendship and all the funs we have during the time we worked together in the project. Finally, I would like to thank my family and my friends for their love and spiritually supporting me throughout my research, my writing, and my whole life. My project was funded by The Danish International Development Agency (DANIDA), Ministry Affairs of Foreign Denmark, iAQUA project. 4 SUMMARY The striped catfish Pangasianodon hypophthalmus is one of the most important species in terms of both economy and physiology. The overall objective of this study is to provide input to the assessment of the effects of elevated temperature and/or hypoxia on the respiratory organs of air-breathing catfish Pangasionodon hypophthalmus by implementing stereological methods to reveal plasticity. This research will play a key role in a better understanding the capacity for adaptation of air-breathing fish to temperature increases. Four main projects were investigated in this study. In the first project, the disturbances in blood gasses and haematological parameters, caused by the three different anaesthetics, commonly used in aquaculture during transport as well as for surgical procedures, were investigated during recovery in Pangasianodon hypophthalmus. We found that these parameters were normalized within 24h and this was the first indication that P. hypophthalmus is unusual among air-breathing fish with its strong capacity for acid-base regulation. In addition, this study demonstrated that this species lacks the β-adrenergic swelling responses in red blood cells. In the second project, by applying vertical sections in stereology, gill morphometrics of P. hypophthalmus exposed to the average Mekong river present temperature (27°C) as well as to a constant 6°C elevation were investigated. These temperature treatments were combined with normoxic and hypoxic oxygen levels. We found strong plasticity in gills lamellar surface areas (SA), with highest SA under elevated temperature and hypoxia whereas almost eliminated. This plasticity was due to proliferation of cell mass between the secondary lamellae (ILCM), which was thus most developed in the normoxic low temperature group. Further, the diffusion distance from water to blood (HM) was thinnest (approximately 1.0µm) in the fish exposed to hypoxia and high temperature. At their largest, the gills of this species are on a weight specific basis similar to active water-breathers, such as trout, but with significantly shorter HM. This is the first documentation of ILCM in catfish and is similar to that found in cyprinids and seems to present support for the osmo-respiratory compromise phenomenon. In this study it was also demonstrated for the first time that this species grows much faster at higher than present temperatures, with an 8-fold higher growth rate at 33°C than 27°C. The third project, we examined the development of the gill and air-breathing organ (ABO) SA and HM with body size. By calculating the anatomical diffusion factor, it was possible to evaluate the importance of gills and ABO for oxygen uptake and to make 5 interspecies comparisons. Here I found that the ADF of the gills is high (comparable to an active water-breather such as rainbow trout), even with fully developed ILCM. Further, when required, the gills can change rapidly (<20h) elevating ADF by a factor 3. In addition, the dimensions (respiratory SA, volume) of respiratory organs scaled with body mass, with the scaling slope for gills being in the range found for active water-breathing fish and with the swim bladder scaling as a mammalian lung. It has been argued that there is a compromise between gas exchange and ion regulation functions in fish gills. In the fourth project, we exposed the fish in two different oxygen levels (hypoxia and hyperoxia) to induce gill remodelling and to test whether such branchial remodelling affects the rate of acid/base regulation in response to aquatic hypercapnia in P. hypophthalmus. We found that there was no difference in the rate of acid-base regulation in these two groups, and suggest that despite its well-developed gills that there is a functional separation in this species occurring where the respiratory gill surfaces do not function in ion exchange. 6 Chapter 1: INTRODUCTION 1.1. Oxygen requirement and adaptive mechanisms of fish to hypoxia Climate change, hypoxia, and its effects It has been predicted that the environmental temperature is increasing as a result of global climate change (IPPC, 2014). It is clear that the projected increase in environmental temperature may lead to negative effects on fish populations as a result of disturbances on fish physiology, metabolism, immune functionality, reproductive capacity, behavior, growth performance, or mortality (Watts et al., 2001; Cnaani, 2006; Dalvi el al., 2009; khoshnevis Yazdi and Shakouri, 2010; Singh et al., 2013; Reid et al., 2015). Temperature elevation causes a reduction in oxygen concentration in water (hypoxia), and at the same time an increase in the metabolic demand for oxygen by living organisms, which has been argued to lead to aerobic scope reductions. These are the features underlying the oxygen- and capacity-limited thermal tolerance (OCLTT) hypothesis, which proposes that oxygen delivery is the key mechanism underlying the negative effects of increased temperatures in aquatic organisms (Pörtner, 2001; Pörtner and Farrell, 2008; Pörtner, 2010). It has also been noted that elevated temperature interacting with hypoxia could result in larger effects on organism performance (McBryan et al., 2013). Tropical regions are predicted to be seriously affected by the climate change. Indeed, there are more than 7000 freshwater fish species living in tropical regions, and it has been argued that since these animals are likely stenothermal and since they already live closer to their upper thermal limit, they may actually be the most vulnerable (Nelson, 1994; Tewksbury et al., 2008). Besides, tropical areas are known for significant fluctuations in oxygen content in the water, where water can be richly oxygenated in wet seasons and severely hypoxic or even anoxic in dry season (Welcomme, 1979; Lucas and Baras, 2008; Nguyễn Lâm Anh, 2016). In addition, as a result of primary production in organically rich water with poor mixing, oxygen can fluctuate on a dual basis from deep hypoxia at night to hyperoxia during the day. Fish adaptive mechanisms to hypoxia Fish have developed a variety of adaptive mechanisms in their physiology, morphology, or behaviour to cope with hypoxia in the environment (Wu, 2002; McBryan et al., 2013). Firstly, fish have some biochemical and physiological responses to maintain oxygen delivery, such as increasing gill ventilation to increase water flow over the gills, increase gill perfusion (Randall, 1970, 1982; Wu, 2002), increase the amount of RBC (Randall, 1982; Soldatov, 1996; Paper 1), or increase affinity for O2 in haemoglobin (Randall, 1982; Val et al., 7 1995; Damsgaard et al., 2015b). The fish may reduce their swimming activities to reduce oxygen demand and conserve energy expenditure under hypoxia (Schurmann and Steffensen, 1994). Besides, numerous fish have been found with some mechanisms to increase capacity for oxygen uptake under hypoxic conditions by changing their respiratory structures (discussed in the following section). During long-term exposure to hypoxia, many fish species have formed an adaptive behaviour that they can migrate to more oxygenated waters or move to the surface of the water for aquatic surface respiration or aerial respiration when the water becomes hypoxic (Lewis, 1970; Petersen and Petersen, 1990; Wannamaker and Rice, 2000; Kramer and Mehegan, 1981; Chapman et al., 1995). Air-breathing is one of the adaptive responses of fish to aquatic hypoxia (Johansen et al., 1970; Randall et al., 1981; Graham, 1997). Many species especially in tropical areas have evolved a transition from aquatic to aerial respiration (Graham, 1997). When the gills of fish fail to meet oxygen requirement for their metabolic ... r-breathing fish have to date, been performed on Amazonian species from extremely ion-poor water (Shartau and Brauner, 2014). These species exhibit low capacities for pH regulation, and possibly the capacity for pH regulation was selected against due to the high metabolic cost of ionoregulation under these conditions (Heisler, 1982; Brauner et al., 2004b; Harter et al., 2014). In contrast, air-breathing species inhabiting environments with higher ion levels, such as P. hypophthalmus in the Mekong Delta where ion levels such as calcium sodium and chloride are 10-50 times higher, may have retained the ability for pH regulation, as it is less metabolically costly to pH compensate in response to hypercapnia under these environmental conditions (Damsgaard et al., 2015; Gam et al., 2017; Thinh et al., unpublished data). Acknowledgements This study was funded by The Danish International Development Agency (DANIDA), Danish Ministry of Foreign Affairs, iAQUA project. 116 References Baddeley, A.J., Gundersen, H.J.G. and Cruz Orive, L.M., 1986. Estimation of surface area from vertical sections. J Micros, 142(3): 259-276. Boutilier, R.G., Iwama, G.K., Heming, T.A. and Randall, D.J., 1985. The apparent pK of carbonic acid in rainbow trout blood plasma between 5 and 15 C. Respir Physiol, 61(2): 237-254. Bradshaw, J.C., Kumai, Y., and Perry, S.F., 2012. The effects of gill remodeling on transepithelial sodium fluxes and the distribution of presumptive sodium-transporting ionocytes in goldfish (Carassius auratus). J Comp Physiol B, 182:351–366. Brauner, C.J. and Baker, D.W., 2009. Patterns of acid–base regulation during exposure to hypercarbia in fishes. In Cardio-respiratory control in vertebrates (pp. 43-63). Springer Berlin Heidelberg. Brauner, C.J., Matey, V., Wilson, J.M., Bernier, N.J. and Val, A.L., 2004a. Transition in organ function during the evolution of air-breathing; insights from Arapaima gigas, an obligate air-breathing teleost from the Amazon. J Exp Biol, 207(9): 1433-1438. Brauner, C.J., Wang, T., Wang, Y., Richards, J.G., Gonzalez, R.J., Bernier, N.J., Xi, W., Patrick, M. and Val, A.L., 2004b. Limited extracellular but complete intracellular acid-base regulation during short-term environmental hypercapnia in the armoured catfish, Liposarcus pardalis. J Exp Biol, 207(19): 3381-3390. da Costa, O.T., Pedretti, A.C.E., Schmitz, A., Perry, S.F. and Fernandes, M.N., 2007. Stereological estimation of surface area and barrier thickness of fish gills in vertical sections. J Micros, 225(1): 1-9. Damsgaard, C., Tuong, D.D., Thinh, P.V., Wang, T. and Bayley, M., 2015. High capacity for extracellular acid–base regulation in the air-breathing fish Pangasianodon hypophthalmus. J Exp Biol, 218(9): 1290-1294. Evans, D.H., Piermarini, P.M. and Choe, K.P., 2005. The multifunctional fish gill: dominant site of gas exchange, osmoregulation, acid-base regulation, and excretion of nitrogenous waste. Physiol Rev, 85(1): 97-177. 117 Fernandes, M.N. and Perna-Martins, S.A., 2001. Epithelial gill cells in the armored catfish, Hypostomus CF. plecostomus (Loricariidae). Revista Brasileira de Biologia, 61(1), pp.69- 78. Fu, S.J., Brauner, C.J., Cao, Z.D., Richards, J.G., Peng, J. L., Dhillon, R., & Wang, Y.X., 2011. The effect of acclimation to hypoxia and sustained exercise on subsequent hypoxia tolerance and swimming performance in goldfish (Carassius auratus). J Exp Biol, 214(12): 2080-2088. Gam, L.T.H., Jensen, F.B., Damsgaard, C., Huong, D.T.T., Phuong, N.T. and Bayley, M., 2017. Extreme nitrite tolerance in the clown knifefish Chitala ornata is linked to up-regulation of methaemoglobin reductase activity. Aquatic Toxicol, 187: 9-17. Gonzalez, R. and Mcdonald, D.G., 1994. The relationship between oxygen uptake and ion loss in fish from diverse habitats. J Exp Biol, 190(1): 95-108. Gonzalez, R.J. and McDonald, D.G., 1992. The relationship between oxygen consumption and ion loss in a freshwater fish. J Exp Biol, 163(1): 317-332. Goss, G.G., Laurent, P. and Perry, S.F., 1992. Evidence for a morphological component in acid- base regulation during environmental hypercapnia in the brown bullhead (Ictalurus nebulosus). Cell Tissue Res, 268(3): 539-552. Graham, J.B. ed., 1997. Air-breathing fishes: evolution, diversity, and adaptation. Academic Press.Sollid, J., Weber, R.E. and Nilsson, G.E., 2005. Temperature alters the respiratory surface area of crucian carp Carassius carassius and goldfish Carassius auratus. J Exp Biol, 208(6): 1109-1116. Gundersen, H.J.G., Bagger, P., Bendtsen, T.F., Evans, S.M., Korbo, L.X.M.N., Marcussen, N., Møller, A., Nielsen, K., Nyengaard, J.R., Pakkenberg, B. and Sørensen, F.B., 1988. The new stereological tools: disector, fractionator, nucleator and point sampled intercepts and their use in pathological research and diagnosis. Apmis, 96(7‐12): 857-881. Harter, T.S., Shartau, R.B., Baker, D.W., Jackson, D.C., Val, A.L. and Brauner, C.J., 2014. Preferential intracellular pH regulation represents a general pattern of pH homeostasis during acid–base disturbances in the armoured catfish, Pterygoplichthys pardalis. J Comp Physiol B, 184(6): 709-718. 118 Heisler, N.O.R.B.E.R.T., 1982. Intracellular and extracellular acid-base regulation in the tropical fresh-water teleost fish Synbranchus marmoratus in response to the transition from water breathing to air breathing. J Exp Biol, 99(1): 9-28. Howard, C.V. and Reed, M.G., 1998. Unbiased Stereology. Three-dimensional Measurement in Microscopy. Bios Scientific Publishers, Oxford, U.K Hvas, M., Damsgaard, C., Jensen, F.B. and Bayley, M., 2016. The effect of environmental hypercapnia and size on nitrite toxicity in the striped catfish (Pangasianodon hypophthalmus). Aquatic Toxicol, 176: 151-160. Jensen, E.B., Gundersen, H.J.G. and Østerby, R., 1979. Determination of membrane thickness distribution from orthogonal intercepts. J Micros, 115(1): 19-33. McBryan, T.L., Healy, T.M., Haakons, K.L. and Schulte, P.M., 2016. Warm acclimation improves hypoxia tolerance in Fundulus heteroclitus. J Exp Biol, 219(4): 474-484. Michel, R.P. and Cruz‐Orive, L.M., 1988. Application of the Cavalieri principle and vertical sections method to lung: estimation of volume and pleural surface area. J Mmicros, 150(2): 117-136. Mitrovic, D. and Perry, S.F., 2009. The effects of thermally induced gill remodeling on ionocyte distribution and branchial chloride fluxes in goldfish (Carassius auratus). J Exp Biol, 212(6): 843-852. Moron, S.E., Andrade, C.A.D. and Fernandes, M.N., 2009. Response of mucous cells of the gills of traíra (Hoplias malabaricus) and jeju (Hoplerythrinus unitaeniatus) (Teleostei: Erythrinidae) to hypo-and hyper-osmotic ion stress. Neotropic Ichthyol, 7(3), pp.491-498. Moron, S.E., Oba, E.T., De Andrade, C.A. and Fernandes, M.N., 2003. Chloride cell responses to ion challenge in two tropical freshwater fish, the erythrinids Hoplias malabaricus and Hoplerythrinus unitaeniatus. J Exp Zool A: Ecol Gen Physiol, 298(2): 93-104. Nilsson, G.E., Dymowska, A. and Stecyk, J.A., 2012. New insights into the plasticity of gill structure. Res Physiol Neurobiol, 184(3): 214-222. Nilsson, S., 1986. Control of gill blood flow. In Fish Physiology: Recent Advances (pp. 86-101). Springer Netherlands. 119 Olson, K.R. and Fromm, P.O., 1973. Mercury uptake and ion distribution in gills of rainbow trout (Salmo gairdneri): tissue scans with an electron microprobe. J Fisher Board Can, 30(10): 1575-1578. Perry, S.F. and Laurent, P., 1993. Environmental effects on fish gill structure and function. In Fish ecophysiology (pp. 231-264). Springer Netherlands. Perry, S.F., Fletcher, C., Bailey, S., Ting, J., Bradshaw, J., Tzaneva, V. and Gilmour, K.M., 2012. The interactive effects of exercise and gill remodeling in goldfish (Carassius auratus). J Comp Physiol B, 182(7): 935-945. Phuong, L.M., Damsgaard, C., Ishimatsu, A., Wang, T. and Bayley, M., 2017a. Recovery of blood gases and haematological parameters upon anaesthesia with benzocaine, MS-222 or Aqui- S in the air-breathing catfish Pangasianodon hypophthalmus. Ichthyol Res, 64(1): 84-92. Phuong, L.M., Huong, D.T.T., Nyengaard, J.R. and Bayley, M., 2017b. Gill remodeling and growth rate of striped catfish Pangasianodon hypophthalmus under impacts of hypoxia and temperature. Comp Biochem Physiol A, 203: 288-296. Randall, D.J., 1981. The evolution of air breathing in vertebrates. Cambridge University Press. Randall, D.J., Baumgarten, D. and Malyusz, M., 1972. The relationship between gas and ion transfer across the gills of fishes. Comp Biochem Physiol A, 41(3): 629-637. Sardella, B.A. and Brauner, C.J., 2007. The osmo-respiratory compromise in fish: the effects of physiological state and the environment. Fish Respirir Environ, 147-165. Shartau, R.B. and Brauner, C.J., 2014. Acid–base and ion balance in fishes with bimodal respiration. J Fish Biol, 84(3): 682-704. Sinha, A.K., Matey, V., Giblen, T., Blust, R., and Boeck, G.D., 2014. Gill remodeling in three freshwater teleosts in response to high environmental ammonia. Aquatic Toxicol, 155: 166– 180. Soivio, A.N.T.T.I., Nynolm, K.E.I.J.O. and Westman, K., 1975. A technique for repeated sampling of the blood of individual resting fish. J Exp Biol, 63(1): 207-217. 120 Sollid, J., De Angelis, P., Gundersen, K. and Nilsson, G.E., 2003. Hypoxia induces adaptive and reversible gross morphological changes in crucian carp gills. J Exp Biol, 206(20): 3667- 3673. Sollid, J., Weber, R.E. and Nilsson, G.E., 2005. Temperature alters the respiratory surface area of crucian carp Carassius carassius and goldfish Carassius auratus. J Exp Biol, 208(6): 1109- 1116. Stewart, P.A., 1978. Independent and dependent variables of acid-base control. Respir physiol, 33(1): 9-26. Wood, C.M. and Randall, D.J., 1973. The influence of swimming activity on sodium balance in the rainbow trout (Salmo gairdneri). J Comp Physiol A: Neuroethol Sens Neural Behav Physiol, 82(3): 207-233. Wood, C.M. and Randall, D.J., 1973. The influence of swimming activity on water balance in the rainbow trout (Salmo gairdneri). J Comp Physiol A: Neuroethol Sens Neural Behav Physiol, 82(3): 257-276. 121 Chapter 6: Conclusions and Perspectives 6.1 Conclusions In anaesthesia experiment with striped catfish, the all three anaesthetics effectively immobilized the fish and reduced responses to tactile stimulation to a level where transport or minor surgical procedures could be performed, but they also caused significant changes with similar patterns on gas and haematological parameters which were generally normalized within 24 h. Especially, the main findings from this study showed that P. hypophthalmus is unusual among air-breathing fish with its strong capacity for acid-base regulation after a disturbance caused by the three anaesthetics. This dissertation also documents the high plastic responses of P. hypophthalmus gills to the different temperatures and hypoxic levels, with highest SA under high temperature and hypoxia whereas almost eliminated in lower temperature and normoxia due to the proliferation of cell mass between the secondary lamellae (ILCM). Moreover, the harmonic mean diffusion distance was found thinnest (around 1.0µm) in the fish exposed to hypoxia and high temperature. The findings of ILCM in P. hypophthalmus gills have revealed the first documentation of ILCM in catfish, which is similar to what have been found in cyprinids and seems to present support for the osmo- respiratory compromise phenomenon. By measuring morphometrics (respiratory surface area and harmonic mean water- or air- blood diffusion distance) of respiratory organs of P. hypophthalmus, this thesis has morphologically demonstrated that this species has both well-developed gills and highly vascularized swim bladder indicating high capacity for both aquatic and aerial gas exchange. It was found that the ADF of the gills is high (comparable to an active water-breather such as rainbow trout), even with fully developed ILCM. Further, when required, the gills can change rapidly (<20h) elevating ADF by a factor 3. In addition, we have found there is a scaling relationship between these metrics with fish body mass, with the scaling slope for gills being in the range found for active water-breathing fish and with the swim bladder scaling as a mammalian lung. It was also demonstrated that the prolonged exposure to hypoxia has been found to cause a reduction in fish growth performance due to the energy cost for physiological and biochemical adjustments of the fish to these conditions. The results in this dissertation show that hypoxia 122 negatively impacted on growth performance of P. hypophthalmus, possibly due to the extra respiratory cost for air-breathing of the fish; whereas, elevated temperature dramatically drive the growth of this species, with fish cultured at 33ᵒC growing approximately 8 fold faster compared to those at 27ᵒC. 6.2 Perspectives The findings from growth performance of striped catfish under the effects of high temperature and/or hypoxia would illustrate that P. hypophthalmus still performs well under the effect of global warming when the predicted temperature to be increased 5ᵒC over the next century. In addition, the aquaculture production of P. hypophthalmus would be improved if improving the rearing conditions such as alleviating hypoxia by supplying oxygen or increasing water exchange in the culture system. From this thesis, moreover, the question remains as to why striped catfish reduces its branchial ADF in normoxic water. Reducing the respiratory SA by adding the ILCM barrier may help prevent ion loss or unwanted water uptake and therefore reduce ion-regulatory energy consumption. However, further research is needed to confirm this as we could see no evidence of mitochondrial rich cells on the secondary lamellae in the present study. Also, the mechanism as well as the speed with which ILCM can be increased or reduced should be examined and whether there are vascular shunts within the gills, or whether there is some other mechanism modulating the leakiness of lamellar epithelial membrane in this species. 123 APPENDICE Fig. S1. Bilogarithmic plots of lamellar SA in relation to gill arch mass of Pangasianodon hypophthalmus. * indicates data from Phuong et al. (2017) of fish with no ILCM; # indicates potential lamella SA from first gill arch in this study. The equation for the regression line (Black line) for the whole data set is Y=0.834X +3.75 and R2=0.845. For the data from Phuong et al., (2017) the regression line (red dashed line) is given by Y=0.820X+3.71 and R2=0.705.
File đính kèm:
the_effects_of_elevated_temperature_and_hypoxia_on_the_respi.pdf
Tóm tắt lận án - Tiếng Việt - Lê Mỹ Phương.pdf
Tóm tắt luận án-Tiếng Anh - Lê Mỹ Phương.pdf
Trang thông tin về luận án - Lê Mỹ Phương.docx
Trang thông tin về luận án - Tiếng Anh - Lê Mỹ Phương.docx