Electrospinning of α-Fe2O3 and ZnFe2O4 nanofibers loaded with reduced graphene oxide (rgo) for H2S gas sensing application
Recently, one dimension (1D) nanostructures including nanowires (NWs),
nanorods (NRs), nanotubes (NTs), and nanofibers (NFs) have attracted much
attention for a wide application including optical catalysis, electronic devices,
optoelectronic devices, storage devices, and gas sensors due to their high surface-tovolume ratio [1]. Especially, NFs are used in many fields such as catalysis, sensor,
and energy storage because of their outstanding properties like large surface area-tovolume ratio and flexible surface functionalities [1,2]. There are several approaches
for NFs fabrication, for example, drawing, template, phase separation, selfassembly, and electrospinning [3–5], among which electrospinning is a simple, costeffective and versatile method for NFs production [1,3–6].
Regarding gas-sensing applications, semiconductor metal oxides (SMO) NFs
sensors have a lot of promise due to their advantages of SMO materials, e.g. low
cost, simple fabrication, and high compatibility with microelectronic processing [7–
10]. Furthermore, NFs consist of many nanograins, therefore, grain boundaries are
large, surface-to-volume ratio is very high, and gases easily diffuse along grain
boundaries. As a result, an exceptionally high response was observed in SMO NFs
gas sensors by electrospinning [11,12]. Among various SMO NFs prepared by
electrospinning, α-Fe2O3 has become a potential gas-sensing material because of its
low cost and thermal stability and ability to detect many gases such as NO2, NH3,
H2S, H2, and CO [13,14]. Besides, zinc ferrite ZnFe2O4 (ZFO), a Fe2O3-based
ternary spinel compounds, has been a promising material for detecting gases thanks
to its good chemical and thermal stability, low toxicity, high specific surface area
and excellent selectivity [15–18].
Tóm tắt nội dung tài liệu: Electrospinning of α-Fe2O3 and ZnFe2O4 nanofibers loaded with reduced graphene oxide (rgo) for H2S gas sensing application
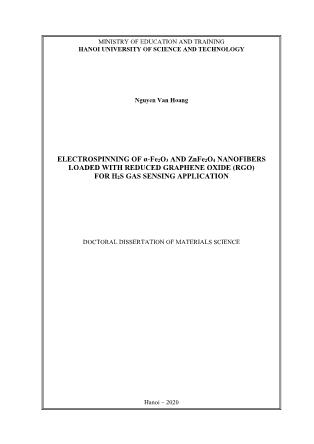
MINISTRY OF EDUCATION AND TRAINING HANOI UNIVERSITY OF SCIENCE AND TECHNOLOGY Nguyen Van Hoang ELECTROSPINNING OF α-Fe2O3 AND ZnFe2O4 NANOFIBERS LOADED WITH REDUCED GRAPHENE OXIDE (RGO) FOR H2S GAS SENSING APPLICATION DOCTORAL DISSERTATION OF MATERIALS SCIENCE Hanoi – 2020 MINISTRY OF EDUCATION AND TRAINING HANOI UNIVERSITY OF SCIENCE AND TECHNOLOGY Nguyen Van Hoang ELECTROSPINNING OF α-Fe2O3 AND ZnFe2O4 NANOFIBERS LOADED WITH REDUCED GRAPHENE OXIDE (RGO) FOR H2S GAS SENSING APPLICATION Major: Materials Science Code: 9440122 DOCTORAL DISSERTATION OF MATERIALS SCIENCE SUPERVISOR: PROF. PhD. NGUYEN VAN HIEU Hanoi – 2020 DECLARATION OF AUTHORSHIP This dissertation has been written in the basic of my researches carried out at Hanoi University of Science and Technology, under the supervision of Prof. PhD. Nguyen Van Hieu. All the data and results in the thesis are true and were agreed to use in my thesis by co-authors. The presented results have never been published by others. . Hanoi, 16th January 2020 Supervisor PhD. Student Prof. PhD. Nguyen Van Hieu Nguyen Van Hoang ACKNOWLEDGMENTS First, I would like to express my deep gratitude to my supervisor, Prof. Nguyen Van Hieu, for his devotion and inspiring supervision. I would like to thank him for all his advice, support and encouragement throughout my postgraduate course. I am grateful to Assoc. Prof. PhD. Nguyen Duc Hoa, Assoc. Prof. PhD. Nguyen Van Duy, PhD. Dang Thi Thanh Le, PhD. Chu Manh Hung, and PhD. Nguyen Van Toan for their useful help, suggestions and comments. I also would like to express my special thanks to PhD and Master Students at iSensors Group for their support and shared cozy working environment during my PhD course. I am thankful to the leaders and staffs of International Training Institute for Materials Science (ITIMS), Graduate School for their help and given favorable working conditions. I would like to thank my colleagues at Department of Materials Science and Engineering at Le Quy Don Technical University for their support during my PhD course. I gratefully acknowledge the fund from Vietnam National Foundation for Science and Technology Development (NAFOSTED) under code 103.02-2017.25 and the 911 Scholarship of Ministry of Education and Training for the financial support for my research. Last but not least, I am deeply thankful to my family for their endless love and unconditional support. Without them, the work would have been impossible. PhD. Student Nguyen Van Hoang i CONTENTS CONTENTS ................................................................................................................ i ABBREVIATIONS AND SYMBOLS ...................................................................... v LIST OF TABLES ................................................................................................... vii LIST OF FIGURES ................................................................................................. viii INTRODUCTION ...................................................................................................... 1 CHAPTER 1. OVERVIEW ON SMO NFs AND THEIR LOADING WITH RGO FOR GAS-SENSING APPLICATION ...................................................................... 6 1.1. Electrospinning for NFs fabrication.............................................................. 6 1.1.1. Background on electrospinning.............................................................. 6 1.1.2. Processing – structure relationships of electrospun NFs ....................... 7 1.2. NFs for gas-sensing application .................................................................. 10 1.2.1. Electrospun SMO NFs for gas-sensing application ............................. 10 1.2.2. Electrospun SMO NFs for H2S gas-sensing application ...................... 13 1.2.2.1. H2S gas .......................................................................................... 13 1.2.2.2. Electrospun SMO NFs for H2S gas-sensing application ............... 13 1.3. NFs loading with RGO for gas-sensing application ................................... 14 1.3.1. Overview on RGO and its application in gas-sensing field ................. 14 1.3.1.1. Overview on RGO ......................................................................... 14 1.3.1.2. RGO in gas-sensing application .................................................... 17 1.3.2. RGO-loaded SMO NFs in gas-sensing applications ............................ 19 1.3.2.1. RGO-loaded SMO gas sensor ....................................................... 19 1.3.2.2. RGO-loaded SMO NFs gas sensor ................................................ 22 ii 1.4. Gas-sensing mechanism .............................................................................. 24 1.4.1. Gas-sensing mechanism of SMO NFs ................................................. 24 1.4.2. Gas-sensing mechanism of RGO-loaded SMO NFs ............................ 25 1.4.3. H2S gas-sensing mechanism of SMO NFs and their loading with RGO.. ............................................................................................................. 27 Conclusion of chapter 1 ............................................................................................ 28 CHAPTER 2. EXPERIMENTAL APPROACH ...................................................... 29 2.1. Synthesis ..................................................................................................... 29 2.1.1. RGO preparation .................................................................................. 29 2.1.2. α-Fe2O3 NFs preparation ...................................................................... 30 2.1.3. ZFO NFs preparation ........................................................................... 31 2.1.4. Preparation of α-Fe2O3, ZFO NFs loading with RGO ......................... 32 2.2. Characterization Techniques ....................................................................... 32 2.2.1. Raman spectroscopy ............................................................................. 32 2.2.2. Thermal analysis .................................................................................. 33 2.2.3. X-ray diffraction ................................................................................... 33 2.2.4. SEM and EDX ...................................................................................... 34 2.2.5. TEM and SAED ................................................................................... 34 2.3. Gas-sensing measurement ........................................................................... 35 Conclusion of chapter 2 ............................................................................................ 36 CHAPTER 3. α-Fe2O3 NFs AND THEIR LOADING WITH RGO FOR H2S GAS- SENSING APPLICATION ...................................................................................... 37 3.1. Introduction ................................................................................................. 37 3.2. H2S gas sensors based on α-Fe2O3 NFs ...................................................... 39 3.2.1. Morphologies and structures of α-Fe2O3 NFs ...................................... 39 iii 3.2.2. H2S gas-sensing properties of α-Fe2O3 NFs sensors ............................ 46 3.2.2.1. Effects of operating temperature ................................................... 46 3.2.2.2. Effects of solution contents ........................................................... 48 3.2.2.3. Effects of annealing temperature and electrospinning time .......... 50 3.2.2.4. Selectivity and stability ................................................................. 53 3.3. H2S gas sensors based on α-Fe2O3 NFs loaded with RGO ......................... 54 3.3.1. Morphologies and structures of α-Fe2O3 NFs loaded with RGO ......... 54 3.3.2. H2S gas-sensing properties of RGO-loaded α-Fe2O3 NFs sensors ...... 58 3.3.2.1. Effects of RGO contents ................................................................ 58 3.3.2.2. Effects of working temperature ..................................................... 61 3.3.2.3. Effects of annealing temperatures ................................................. 62 3.3.2.4. Selectivity and stability ................................................................. 64 Conclusion of chapter 3 ............................................................................................ 65 CHAPTER 4. ZFO NFs AND THEIR LOADING WITH RGO FOR H2S GAS- SENSING APPLICATION ...................................................................................... 66 4.1. Introduction ................................................................................................. 66 4.2. H2S gas sensors based on ZFO NFs ............................................................ 68 4.2.1. Microstructure characterization ........................................................... 68 4.2.2. Gas-sensing properties ......................................................................... 74 4.2.2.1. Effects of the operating temperature ............................................. 74 4.2.2.2. Effects of the annealing temperature ............................................. 76 4.2.2.3. Effects of annealing time and heating rate .................................... 79 4.2.2.4. Selectivity and stability ................................................................. 81 4.3. H2S gas sensors based on ZFO NFs loaded with RGO .............................. 82 4.3.1. Microstructure characterization ........................................................... 82 iv 4.3.2. Gas-sensing properties ......................................................................... 86 4.3.2.1. Effects of RGO contents ................................................................ 86 4.3.2.2. Effects of operating temperature ................................................... 88 4.3.2.3. Effects of annealing temperatures ................................................. 89 4.3.2.4. Selectivity, stability and RH effects .............................................. 91 Conclusion of chapter 4 ............................................................................................ 94 CONCLUSIONS AND RECOMMENDATIONS ................................................... 95 LIST OF PUBLICATIONS ...................................................................................... 97 REFERENCES ......................................................................................................... 98 APPENDIX ............................................................................................................ 117 v ABBREVIATIONS AND SYMBOLS Number Abbreviations and symbols Meaning 1 1D One Dimension 2 2D Two Dimension 3 CVD Chemical Vapor Deposition 4 DI Deionized Water 5 DL Detection Limit 6 DMF Dimethylformamide 7 DTG Derivative Thermogravimetric 8 EDX Energy Dispersive X-ray spectroscopy 9 FE-SEM Field Emission Scanning Electron Microscope 10 FFT Fast Fourier Transform 11 GO Graphene Oxides 12 GP Graphene 13 HRTEM High Resolution Transmission Electron Microscope 14 IUPAC International Union of Pure and Applied Chemistry 15 JCPDS Joint Committee on Powder Diffraction Standards 16 NFs Nanofibers 17 NPs Nanoparticles 18 NRs Nanorods 19 NSs Nanosheets 20 NTs Nanotubes 21 NWs Nanowires 22 ppb Parts Per Billion 23 ppm Parts Per Million 24 PVA Poly(vinyl alcohol) 25 RGO Reduced Graphene Oxides 26 RH Ambient Relative Humidity 27 RT Room Temperature vi 28 SAED Selected Area Electron Diffraction 29 sccm Standard Cubic Centimeters Per Minute 30 SEM Scanning Electron Microscope 31 SMO Semiconductor Metal Oxides 32 TEM Transmission Electron Microscope 33 TGA Thermogravimetric Analysis 34 WF Work Function 35 XRD X-ray Diffraction 36 ZFO Zinc Ferrite, ZnFe2O4 37 Ra Sensor resistance in dry air 38 Rg Sensor resistance in tested gas 39 S Sensor Response 40 τres Response time 41 τrec Recovery time vii LIST OF TABLES Table 1.1. SMO NFs for gas-sensing application .................................................... 12 Table 2.1. Processing parameter of of α-Fe2O3 NFs, ZFO NFs and their loading with RGO .................................................................................................................. 31 Table 3.1. Different nanostructures of α-Fe2O3 for H2S gas-sensing application ... 38 Table 3.2. α-Fe2O3 loaded with RGO for gas-sensing application .......................... 38 Table 4.1. Different nanostructures of ZFO for H2S gas-sensing application ......... 67 Table 4.2. Comparison of the H2S gas sensitivity of the sensor based on other nanomaterials and nanostructures ............................................................................. 93 Table A3.1. Calculation table of DL to H2S of sensors based on α-Fe2O3 NFs loaded with different contents of RGO from 0 to 1.5 wt% RGO at 350°C. ...................... 117 Table A3.2. Calculation table of DL to H2S of α-Fe2O3 NFs sensors calcined at annealing temperatures from 400°C to 800°C at 350°C. ...................................... 118 Table A3.3. Calculation table of DL to H2S of 1.0 wt.% RGO-loaded α-Fe2O3 NFs sensors calcined at annealing temperatures from 400°C to 800°C at 350°C. ........ 119 Table A4.1. Average nanograin sizes determined by Scherrer formula and integrated intensity of (311) diffraction peak of ZFO-NFs calcined at different conditions. ............................................................................................................... 120 Table A4.2. Response and response-recovery time to 1 ppm H2S gas at the operating temperature of 350°C of the ZFO NFs sensors calcined at different annealing temperatures (400−700°C), annealing time (0.5−48 h), heating rates (0.5−20°C/min), electrospinning time (10−120 min). ............................................ 121 Table A4.3. Calculation table of DL to H2S of the ZFO NFs sensors calcined at the annealing temperature from 400°C to 700°C at 350°C. ......................................... 122 Table A4.4. Calculation table of DL to H2S of the 1 wt% RGO-loaded ZFO NFs sensors calcined at annealing temperatures from 400°C to 700°C at 350°C. ........ 123 viii LIST OF FIGURES Figure 1.1: Schematic diagram of electrospinning method: 1-Collector, 2-As-spun fibers, 3-Precursor solution, 4- Syringe, 5-Needle, 6- DC voltage power supply. .... 7 Figure 1.2. Kind of collectors and needles: (a) plate collector (b) Multiple spinnerets (c) Coaxial spinneret (d) Bi ... inel ferrite: A highly versatile gas sensor” , Mater Lett, Vol. 124, pp. 144–147. [158] S. Ayyappan, G. Paneerselvam, M. P. Antony, and J. Philip (2011), “Structural stability of ZnFe2O4 nanoparticles under different annealing conditions” , Mater Chem Phys, Vol. 128, pp. 400–404. [159] P. Ghosh, A. Mukherjee, M. Fu, S. Chattopadhyay, and P. Mitra (2015), “Influence of particle size on H2 and H2S sensing characteristics of nanocrystalline nickel ferrite” , Phys E Low-dimensional Syst Nanostructures, Vol. 74, pp. 570–575. [160] C. J. Belle, A. Bonamin, U. Simon, J. Santoyo-Salazar, M. Pauly, S. Bégin- Colin, and G. Pourroy (2011), “Size dependent gas sensing properties of spinel iron oxide nanoparticles” , Sensors Actuators, B Chem, Vol. 160, pp. 942–950. [161] A. Katoch, S. W. Choi, J. H. Kim, J. H. Lee, J. S. Lee, and S. S. Kim (2015), “Importance of the nanograin size on the H2S-sensing properties of ZnO- CuO composite nanofibers” , Sensors Actuators, B Chem, Vol. 214, pp. 111– 116. [162] F. Lu, Y. Liu, M. Dong, and X. Wang (2000), “Nanosized tin oxide as the novel material with simultaneous detection towards CO, H2 and CH4” , Sensors Actuators B, Vol. 66, pp. 225–227. [163] S. G. Ansari, S. R. Sainkar, R. N. Karekar, R. C. Aiyer, and S. K. Kulkarni (1997), “Grain size effects on H2 gas sensitivity of thick film resistor using SnO2 nanoparticles”Vol. 295, pp. 271–276. [164] Y.-F. Sun, S.-B. Liu, F.-L. Meng, J.-Y. Liu, Z. Jin, L.-T. Kong, and J.-H. Liu (2012), “Metal Oxide Nanostructures and Their Gas Sensing Properties: A 115 Review” , Sensors, Vol. 12, pp. 2610–2631. [165] G. Korotcenkov (2008), “The role of morphology and crystallographic structure of metal oxides in response of conductometric-type gas sensors” , Mater Sci Eng R Reports, Vol. 61, pp. 1–39. [166] X. Zhou, J. Liu, C. Wang, P. Sun, X. Hu, X. Li, K. Shimanoe, N. Yamazoe, and G. Lu (2015), “Highly sensitive acetone gas sensor based on porous ZnFe2O4 nanospheres” , Sensors Actuators, B Chem, Vol. 206, pp. 577–583. [167] A. F. S. Abu-Hani, S. T. Mahmoud, F. Awwad, and A. I. Ayesh (2017), “Design, fabrication, and characterization of portable gas sensors based on spinel ferrite nanoparticles embedded in organic membranes” , Sensors Actuators B Chem, Vol. 241, pp. 1179–1187. [168] Y. Cao, D. Jia, P. Hu, and R. Wang (2013), “One-step room-temperature solid-phase synthesis of ZnFe2O4 nanomaterials and its excellent gas-sensing property” , Ceram Int, Vol. 39, pp. 2989–2994. [169] Y. Yan, P. Nizamidin, G. Turdi, N. Kari, and A. Yimit (2017), “Room- temperature H2S Gas Sensor Based on Au-doped ZnFe2O4” , Anal Sci, Vol. 33, pp. 945–951. [170] H. Qiao, Z. Xia, Y. Fei, L. Cai, R. Cui, Y. Cai, Q. Wei, and Q. Yao (2017), “Electrospinning combined with hydrothermal synthesis and lithium storage properties of ZnFe2O4-graphene composite nanofibers” , Ceram Int, Vol. 43, pp. 2136–2142. [171] S. Yang, X. Song, P. Zhang, and L. Gao (2015), “Heating-Rate-Induced Porous α ‑ Fe2O3 with Controllable Pore Size and Crystallinity Grown on Graphene for Supercapacitors” , ACS Appl Mater Interfaces, Vol. 7, pp. 75−79. [172] J. M. Chem, P. F. Teh, Y. Sharma, S. Pramana, and M. Srinivasan (2011), “Nanoweb anodes composed of one-dimensional, high aspect ratio, size tunable electrospun ZnFe2O4 nanofibers for lithium ion batteries” , J Mater Chem, Vol. 21, pp. 14999–15008. [173] K. Wu, J. Li, and C. Zhang (2019), “Zinc ferrite based gas sensors: A review” , Ceram Int, Vol. 45, pp. 11143–11157. 116 [174] J. Y. Park, K. Asokan, S. W. Choi, and S. S. Kim (2011), “Growth kinetics of nanograins in SnO2 fibers and size dependent sensing properties” , Sensors Actuators, B Chem, Vol. 152, pp. 254–260. [175] M. Zong, Y. Huang, H. Wu, Y. Zhao, Q. Wang, and X. Sun (2014), “One-pot hydrothermal synthesis of RGO/CoFe2O4 composite and its excellent microwave absorption properties” , Mater Lett, Vol. 114, pp. 52–55. [176] H. Zhang, A. Xie, C. Wang, H. Wang, Y. Shen, and X. Tian (2013), “Novel rGO/α-Fe2O3 composite hydrogel: synthesis, characterization and high performance of electromagnetic wave absorption” , J Mater Chem A, Vol. 1, pp. 8547–8552. [177] S. K. Pandey, K.-H. Kim, and K.-T. Tang (2012), “A review of sensor-based methods for monitoring hydrogen sulfide” , TrAC Trends Anal Chem, Vol. 32, pp. 87–99. [178] A. Mirzaei, S. S. Kim, and H. W. Kim (2018), “Resistance-based H2S gas sensors using metal oxide nanostructures: A review of recent advances” , J Hazard Mater, Vol. 357, pp. 314–331. [179] L. Guo, X. Kou, M. Ding, C. Wang, L. Dong, H. Zhang, C. Feng, Y. Sun, Y. Gao, P. Sun, and G. Lu (2017), “Reduced graphene oxide/α-Fe2O3 composite nanofibers for application in gas sensors” , Sensors Actuators B Chem, Vol. 244, pp. 233–242. [180] Jiwei Deng, Jianmin Ma, L. Mei, Y. Tang, Y. Chen, T. Lv, Z. Xu, and T. Wang (2013), “Porous α-Fe2O3 nanosphere-based H2S sensor with fast response, high selectivity and enhanced sensitivity” , J Mater Chem A, Vol. 1, pp. 12400–12403. [181] Z. U. Abideen, H. W. Kim, and S. S. Kim (2015), “An ultra-sensitive hydrogen gas sensor using reduced graphene oxide-loaded ZnO nanofibers” , Chem Commun, Vol. 51, pp. 15418–15421. [182] S. Ferrari, R. S. Kumar, F. Grinblat, J. C. Aphesteguy, F. D. Saccone, and D. Errandonea (2016), “In-situ high-pressure x-ray diffraction study of zinc ferrite nanoparticles” , Solid State Sci, Vol. 56, pp. 68–72. 117 APPENDIX Table A3.1. Calculation table of DL to H2S of sensors based on α-Fe2O3 NFs loaded with different contents of RGO from 0 to 1.5 wt% RGO at operating temperature of 350°C. Samples RSS rmsnoise Slope DL (ppb) 0 3.66E-05 0.001913113 5.53 1.04 0.5 3.03E-05 0.00174069 6.76 0.78 1 2.40E-05 0.001549193 8.66 0.54 1.5 1.34E-05 0.001157584 2.44 1.42 Figure A3.1. Fitted values of RSS and slope for DL calculation of sensors based on α-Fe2O3 NFs loaded with different contents of RGO of 0 (a, e), 0.5 (b, f), 1.0 (c, g), and 1.5 wt.% (d, h), respectively. The sensors are tested with H2S gas at operating temperature of 350°C. 0 2 4 6 8 10 0.98 1.00 1.02 1.04 1.06 1.08 0.96 0.98 1.00 1.02 1.04 Time (s) 1.5 wt.% RGO@ air & 350 o C Fifth-order polynomial fit RSS = 1.34E-5 1.0 wt.% RGO@ air & 350 o C Fifth-order polynomial fit RSS = 2.4E-5 R e s p . B a s e ( R a /R g ) 0.5 wt.% RGO @ air & 350 o C Fifth-order polynomial fit RSS = 3.03E-5 Pure - Fe 2 O 3 NFs@ air & 350 o C Fifth-order polynomial fit RSS = 3.66E-5 (a) (b) (c) (d) 0.00 0.25 0.50 0.75 1.00 0 5 0 5 10 0 5 10 0 5 10 H 2 S conc. (ppm) 1.5 wt.% RGO@ air & 350 o C Linear Fit Slope = 2.44 1.0 wt.% RGO@ air & 350 o C Linear Fit Slope = 8.66 R e s p . (R a /R g ) 0.5 wt.% RGO @ air & 350 o C Linear Fit Slope = 6.76 Pure - Fe 2 O 3 NFs@ air & 350 o C Linear Fit Slope = 5.53 (f) (g) (h) (i) 118 Table A3.2. Calculation table of DL to H2S of α-Fe2O3 NFs sensors calcined at annealing temperatures from 400°C to 800°C at operating temperature of 350°C. Samples RSS rmsnoise Slope DL (ppb) 400 oC 1.31E-05 0.001144552 4.15 0.83 500 oC 5.12E-06 0.000715542 2.76 0.78 600 oC 3.66E-05 0.001913113 5.53 1.04 700 oC 2.34E-05 0.001529706 4.48 1.02 800 oC 1.77E-05 0.001330413 0.83 4.81 0.00 0.25 0.50 0.75 1.00 0 5 0 5 0 5 0 5 0 5 H 2 S conc. (ppm) Cal. 800 o C@ H 2 S & 350 o C Linear Fit Slope = 0.83 Cal. 700 o C@ H 2 S & 350 o C Linear Fit Slope = 4.48 R e s p . (R a /R g ) Cal. 600 o C@ H 2 S & 350 o C Linear Fit Slope = 5.53 Cal. 500 o C@ H 2 S & 350 o C Linear Fit Slope = 2.76 Fe 2 O 3 Cal. 400 o C@ H 2 S & 350 o C Linear Fit Slope = 4.15 (f) (g) (h) (i) (j) 0 2 4 6 8 10 1.00 1.02 1.04 0.96 0.98 1.02 1.04 1.00 1.02 1.04 1.00 1.02 R e s p . B a s e ( R a /R g ) Time (s) Cal. 800 o C@ air & 350 o C Fifth-order polynomial fit RSS = 1.77E-5 Cal. 700 o C@ air & 350 o C Fifth-order polynomial fit RSS = 2.34E-5 Cal. 600 o C@ air & 350 o C Fifth-order polynomial fit RSS = 3.66E-5 Cal. 500 o C@ air & 350 o C Fifth-order polynomial fit RSS = 5.12E-6 - Fe 2 O 3 Cal. 400 o C@ air&350 o C Fifth-order polynomial fit RSS = 1.31E-5 (a) (b) (c) (d) (e) Figure A3.2. Fitted values of RSS and slope for DL calculation of α-Fe2O3 NFs sensors calcined at various annealing temperatures of 400 (a, f), 500 (b, g), 600 (c, h), 700 (d, i), and 800°C (e, j), respectively. The sensors are tested with H2S gas at operating temperature of 350°C. 119 Table A3.3. Calculation table of DL to H2S of 1.0 wt.% RGO-loaded α-Fe2O3 NFs sensors calcined at annealing temperatures from 400°C to 800°C at operating temperature of 350°C. Samples RSS rmsnoise Slope DL (ppb) 400 oC 1.67E-05 0.001292285 6 0.65 500 oC 1.21E-05 0.0011 3.68 0.90 600 oC 2.40E-05 0.001549193 8.66 0.54 700 oC 4.05E-05 0.002012461 3.12 1.94 800 oC 9.00E-06 0.000948683 0.14 20.33 Figure A3.3. Fitted values of RSS and slope for DL calculation of 1.0 wt.% RGO-loaded α- Fe2O3 NFs sensors calcined at various annealing temperatures of 400 (a, f), 500 (b, g), 600 (c, h), 700 (d, i), and 800°C (e, j), respectively. The sensors are tested with H2S gas at operating temperature of 350°C. 0 2 4 6 8 10 1.00 1.02 1.04 1.00 1.02 1.04 1.06 0.98 1.00 1.02 1.02 1.04 Time (s) Cal. 800 o C@ air & 350 o C Fifth-order polynomial fit RSS = 9.0E-6 Cal. 700 o C@ air & 350 o C Fifth-order polynomial fit RSS = 4.0E-5 R e s p . B a s e ( R a /R g ) Cal. 600 o C@ air & 350 o C Fifth-order polynomial fit RSS = 2.40E-5 Cal. 500 o C@ air & 350 o C Fifth-order polynomial fit RSS = 1.21E-5 1 wt% RGO Cal. 400 o C@ air&350 o C Fifth-order polynomial fit RSS = 1.67E-5 (a) (b) (c) (d) (e) 0.00 0.25 0.50 0.75 1.00 0 5 10 0 5 10 0 5 10 0 5 10 0 5 10 H 2 S conc. (ppm) Cal. 800 o C@ H 2 S & 350 o C Linear Fit Slope = 0.14 Cal. 700 o C@ H 2 S & 350 o C Linear Fit Slope = 3.15 R e s p . (R a /R g ) Cal. 600 o C@ H 2 S & 350 o C Linear Fit Slope = 8.66 Cal. 500 o C@ H 2 S & 350 o C Linear Fit Slope = 3.68 1 wt.% RGO Cal. 400 o C@H 2 S&350 o C Linear Fit Slope = 6.00 (f) (g) (h) (i) (j) 120 Table A4.1. Average nanograin sizes determined by Scherrer formula and integrated intensity of (311) diffraction peak of ZFO-NFs calcined at different conditions. Samples β (FWHM) (radian) Crystallite sizes (nm) Integrated intensity 400 oC 0.62 13.53 20.24 500 oC 0.55 15.16 22.44 600 oC 0.46 18.08 35.39 700 oC 0.35 23.92 84.24 0.5 h 0.51 16.32 24.68 3 h 0.46 18.08 35.39 12 h 0.38 21.82 69.01 48 h 0.36 23.28 108.82 0.5 oC/min 0.46 18.08 35.39 2 oC/min 0.68 12.25 29.19 5 oC/min 0.61 13.63 35.29 20 oC/min 0.55 15.23 46.58 121 Table A4.2. Response and response-recovery time to 1 ppm H2S gas at the operating temperature of 350°C of the ZFO NFs sensors calcined at different annealing temperatures (400−700°C), annealing time (0.5−48 h), heating rates (0.5−20°C/min), electrospinning time (10−120 min). Samples S 1ppm τres. (s) τrec. (s) 400 oC 8.5 47 423 500 oC 61 9 261 600 oC 102 8 206 700 oC 21.8 7 129 0.5 h 34 9 217 3 h 102 8 206 12 h 42.3 5 63 48 h 15.4 6 40 0.5 oC/min 102 8 206 2 oC/min 19.9 7 53 5 oC/min 53.4 5 68 20 oC/min 7.4 12 122 122 Table A4.3. Calculation table of DL to H2S of the ZFO NFs sensors calcined at the annealing temperature from 400°C to 700°C at the operating temperature of 350°C. Samples RSS rmsnoise Slope DL (ppb) 400 oC 1.37E-05 0.00117161 8.32664 0.422 500 oC 3.69E-05 0.00192032 67.70817 0.085 600 oC 3.28E-05 0.001810392 113.96252 0.048 700 oC 4.78E-06 0.002185514 22.34538 0.093 Figure. A4.1. Fitted values of RSS and slope for DL calculation of the sensors based on ZFO NFs calcined at various annealing temperatures of 400 (a,e), 500 (b,f), 600 (c,g), and 700°C (d,h), respectively. The sensors are tested with H2S gas at the operating temperature of 350°C. 0 2 4 6 8 10 1.00 1.02 1.00 1.02 1.00 1.02 1.04 1.06 Time (s) Cal. 700 o C@ air & 350 o C Fifth-order polynomial fit RSS = 4.78E-6 (d) Cal. 600 o C@ air & 350 o C Fifth-order polynomial fit RSS = 3.28E-5 (c) R e s p . B a s e ( R a /R a ) Cal. 500 o C@ air & 350 o C Fifth-order polynomial fit RSS = 3.69E-5 (b) Cal. 400 o C@ air & 350 o C Fifth-order polynomial fit RSS = 1.37E-5 (a) 0.00 0.25 0.50 0.75 1.00 0 10 20 0 50 100 0 25 50 0 5 10 H 2 S conc. (ppm) Cal. 700 o C@ H 2 S & 350 o C Linear Fit Slope = 22.34538 (h) Cal. 600 o C@ H 2 S & 350 o C Linear Fit Slope = 113.96252 (g) R e s p . (R a /R g ) Cal. 500 o C@ H 2 S & 350 o C Linear Fit Slope = 67.70817 (f) Cal. 400 o C@ H 2 S & 350 o C Linear Fit Slope = 8.32664 (e) 123 Table A4.4. Calculation table of DL to H2S of the 1 wt% RGO-loaded ZFO NFs sensors calcined at annealing temperatures from 400°C to 700°C at the operating temperature of 350°C. Samples RSS rmsnoise Slope DL (ppb) 400 oC 5.05E-05 0.002247221 48.94 0.14 500 oC 5.57E-05 0.002360085 86.58 0.08 600 oC 3.28E-05 0.001811077 164.15 0.03 700 oC 5.20E-06 0.002280351 15.25 0.44 Figure A4.2. Fitted values of RSS and slope for DL calculation of the sensors based on 1 wt% RGO loaded ZFO NFs calcined at various annealing temperatures of 400 (a,e), 500 (b,f), 600 (c,g), and 700°C (d,h), respectively. The sensors are tested with H2S gas at the operating temperature of 350°C. 0 2 4 6 8 10 1.04 1.06 1.00 1.02 1.04 0.98 1.00 1.02 0.98 1.00 Time (s) Cal. 700 o C@ air & 350 o C Fifth-order polynomial fit RSS = 5.2E-5 Cal. 600 o C@ air & 350 o C Fifth-order polynomial fit RSS = 3.28E-5 (d) (c) (b) R e s p . B a s e ( R a /R a ) Cal. 500 o C@ air & 350 o C Fifth-order polynomial fit RSS = 5.57E-5 Cal. 400 o C@ air & 350 o C Fifth-order polynomial fit RSS = 5.05E-5 (a) 0.00 0.25 0.50 0.75 1.00 0 10 20 0 50 100 150 0 25 50 75 0 25 50 H 2 S conc. (ppm) Cal. 700 o C@ H 2 S & 350 o C Linear Fit Slope = 15.25 Cal. 600 o C@ H 2 S & 350 o C Linear Fit Slope = 164.15 (h) (g) (f) R e s p . (R a /R g ) Cal. 500 o C@ H 2 S & 350 o C Linear Fit Slope = 86.58 (e) Cal. 400 o C@ H 2 S & 350 o C Linear Fit Slope = 48.94
File đính kèm:
electrospinning_of_fe2o3_and_znfe2o4_nanofibers_loaded_with.pdf
Abstract Thesis Hoang VN.pdf
Abstract Thesis Hoang EN.pdf
Thong tin -VN.pdf
Thong tin-EN.pdf